The greatest molecule
What we particularly appreciate in water
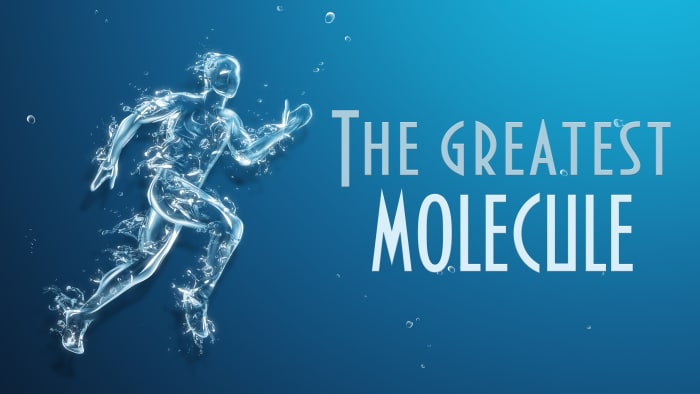
In the 18th century, Antoine Lavoisier ran an electric current through water and discovered two gases in its composition: hydrogen and oxygen.
The formula of the water molecule is H₂O, two hydrogen atoms and one oxygen atom. In this molecule, there is a partial positive charge in the hydrogen atoms, and a partially negative charge in the oxygen atom. This means that molecules can form bonds with each other. These bonds are called hydrogen bonds. It is the small size of the hydrogen atom that allows the strongly polarized molecules of water to come together quite closely to form these bonds. They are not as strong as the atomic bonds within the molecule (covalent bonds), but because of them water molecules are more strongly attracted to each other than the molecules of almost all other substances.
The hydrogen bonds mean that water has a very high specific heat. A lot of energy is required to heat water. Based on the location of oxygen in the periodic table and the boiling temperatures of hydrides (bonds with hydrogen) of elements in the sub-group of oxygen (sulfur, selenium, tellurium), water without hydrogen bonds would boil and freeze at much lower temperatures, similar to hydrogen sulfide which boils at -60 °С and freezes at -82.3 °С.
The hydrogen bonds explain capillary phenomena. We can observe them, for example, when paint rises between the bristles of a brush. Water molecules are so strongly attracted to each other that they overcome the power of gravity. Through the capillaries that run through the tree, water travels from its roots right to the leaves.
Hydrogen bonds give water its high surface tension. This means that water can gather in drops, it can be poured into a cup “with a mound”, and some insects can walk on it as if it were solid ground.
A universal solvent
Hydrogen bonds make water a universal solvent. It dissolves salts, sugars, acids, alkalis and even some gases. These substances are called hydrophilic (water-lovers) because they evaporate easily in water.
On the contrary, fats and oils are hydrophobic. This means that their molecules are not capable of forming hydrogen bonds. So water rejects these molecules, preferring to form bonds within itself. To wash fat off our hands we use soap, which has molecules with hydrophobic and hydrophilic parts. The hydrophobic parts attach to the fat, breaking in into small drops. And with the hydrophilic parts this structure attaches to the flow of water and goes with it down the drain.
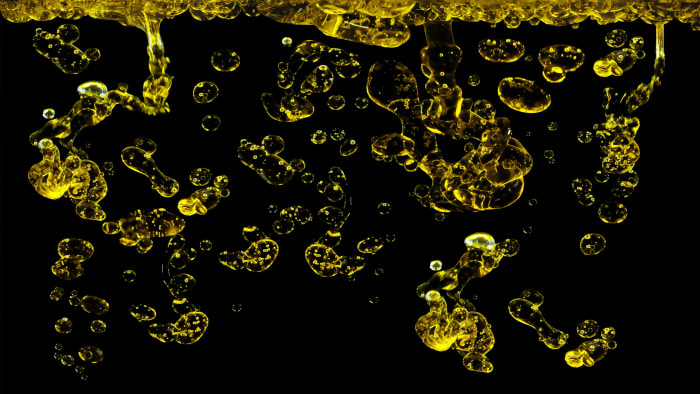
No two snowflakes are identical
Firstly, the smallest change in temperature and humidity affects the form that water molecules freeze in. Secondly, the average snowflake contains 10 quintillion (10 with 18 zeroes) water molecules. And this gives plenty of scope for creativity.
Water is practically the only substance that expands when it is in a solid state. Usually, substances become compacter when they freeze, and take up less volume than liquid forms. But in the case of water, ice is less dense, and so it is light than the same volume of liquid water, which means that ice cubes float in the top layers of our drinks. And, more importantly for living organisms, ice in water bodies also forms on top, and does not let the remaining water freeze. When they freeze, water molecules form an ordered lattice, taking up more space than they required in a liquid state. As a result, ice is 9% less compact than liquid water.
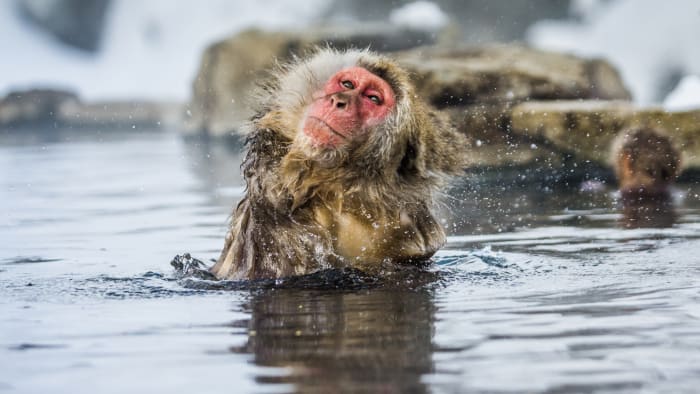
Water is incredibly mobile. It constantly moves across the entire Earth in a cycle of evaporation, condensation and precipitation. Its mobility also affects other organisms in which hydrogen and oxygen components constantly unite and rearrange themselves in biochemical processes.
We not only consume water, but also produce it. Every time when glucose molecules in the body break down, six water molecules form. This reaction takes place in the body of the average person 6 septillion (6 with 24 zeroes) times a day. Nevertheless, we cannot cover our need for water in this way.
How much do we have?
Generally, there is quite a lot of water in the universal, and this is quite logical. The three most widespread elements in the universe are hydrogen, helium and oxygen. But as helium is inert and does not enter into chemical reactions, a compound of the two other elements, i.e. water, is encountered quite frequently. At the same time, all the water on Earth would form a sphere with a diameter of around 1400 km. This is almost 10 times less than the diameter of the Earth itself. Of this volume, only 3% is fresh water. So for each cup of sea water, there is just a little more than a teaspoon of fresh water. 85% of fresh water on the planet is held in glaciers and polar ice. The growth of the population, pollution of water bodies and a number of other factors make it increasingly likely that in the 21st century fresh water may become a deficit, and cost more than petrol.
Fortunately, today we still have the opportunity to raise a glass of water to the most amazing molecule.