Test tube rocket
A test tube takes flight like a rocket!
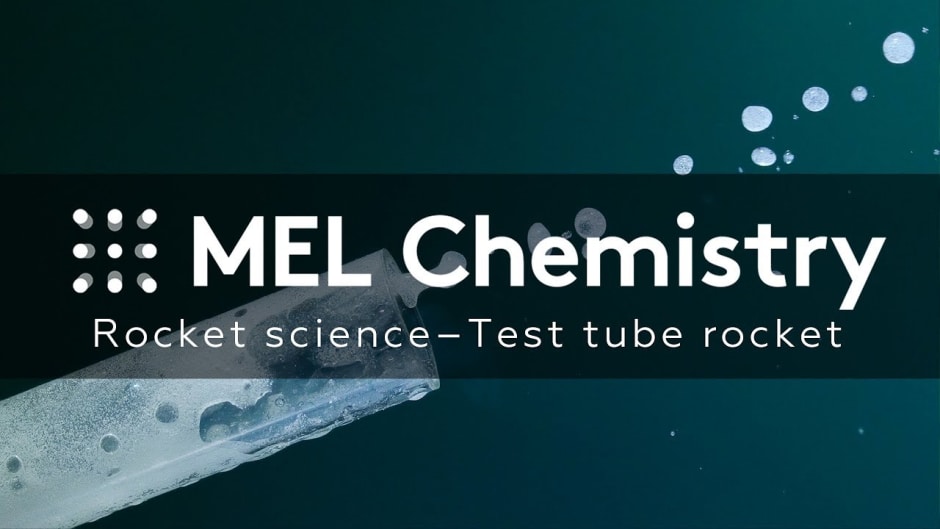
Reagents
Safety
- Put on protective gloves and eyewear.
- Conduct the experiment on the plastic tray.
- Do not allow chemicals to come into contact with the eyes or mouth.
- Keep young children, animals and those not wearing eye protection away from the experimental area.
- Store this experimental set out of reach of children under 12 years of age.
- Clean all equipment after use.
- Make sure that all containers are fully closed and properly stored after use.
- Ensure that all empty containers are disposed of properly.
- Do not use any equipment which has not been supplied with the set or recommended in the instructions for use.
- Do not replace foodstuffs in original container. Dispose of immediately.
- In case of eye contact: Wash out eye with plenty of water, holding eye open if necessary. Seek immediate medical advice.
- If swallowed: Wash out mouth with water, drink some fresh water. Do not induce vomiting. Seek immediate medical advice.
- In case of inhalation: Remove person to fresh air.
- In case of skin contact and burns: Wash affected area with plenty of water for at least 10 minutes.
- In case of doubt, seek medical advice without delay. Take the chemical and its container with you.
- In case of injury always seek medical advice.
- The incorrect use of chemicals can cause injury and damage to health. Only carry out those experiments which are listed in the instructions.
- This experimental set is for use only by children over 12 years.
- Because children’s abilities vary so much, even within age groups, supervising adults should exercise discretion as to which experiments are suitable and safe for them. The instructions should enable supervisors to assess any experiment to establish its suitability for a particular child.
- The supervising adult should discuss the warnings and safety information with the child or children before commencing the experiments. Particular attention should be paid to the safe handling of acids, alkalis and flammable liquids.
- The area surrounding the experiment should be kept clear of any obstructions and away from the storage of food. It should be well lit and ventilated and close to a water supply. A solid table with a heat resistant top should be provided
- Substances in non-reclosable packaging should be used up (completely) during the course of one experiment, i.e. after opening the package.
FAQ and troubleshooting
Indeed, this reagent tends to clump when stored. Use a wooden stick or any other one to break up the clumps.
You can repeat this experiment as many times as you want – you already have all the necessary ingredients at home! Sodium bicarbonate NaHCO3 is ordinary baking soda. And even if you’ve run out of citric acid in your kitchen, don’t rush to a grocery store just yet: use regular vinegar instead of water. Pour 2-3 mL of vinegar into the test tube and perform steps 2–4, now without the citric acid.
When placing the tube in the beaker, position it at a 45o angle.
Step-by-step instructions
The reaction will take place in an aqueous solution.
Dry citric acid and baking soda NaHCO3 do not react with each other, so you can mix them in advance.
To react with each other, the compounds must be dissolved in water.
Dry citric acid and baking soda quickly dissolve in water and react with each other, producing CO2 gas. Eventually, the pressure inside the tube forces the tube to pop off the stopper and launch into the air, almost like a real rocket.
Disposal
Dispose of solid waste together with household garbage. Pour solutions down the sink. Wash with an excess of water.
Scientific description
When we turn the test tube upside down, water begins to dissolve the sodium hydrogen carbonate NaHCO3 and citric acid H3C6H5O7 powders. As these compounds dissolve, some charged particles called ions appear in the solution. One of these ions HCO3-
from NaHCO3 reacts with H+
ions from H3C6H5O7. This ion-exchange reaction produces water H2O
and CO2
gas.
We exploit a similar reaction when baking: sodium hydrogen carbonate reacts with an acidic solution to produce CO2 bubbles and make bread or biscuits fluffier. In our rocket, however, the CO2 accumulates in the test tube, increasing the pressure inside. Eventually, the pressure rises so high that the test tube detaches from the stopper and bursts into the air like a rocket!
The driving force in a space rocket also relies on chemical reactions. A great amount of energy is needed to lift such a huge object, and this is difficult to achieve by merely exchanging ions. A different type of chemical reaction—an oxidation-reduction (redox) reaction is utilized instead. The most well-known oxidation-reduction reaction takes place whenever something burns!
Why does the test tube launch into the air?
The test tube takes flight because of gas formed via a chemical reaction. As the tube is closed securely with a stopper, the gas accumulates there. Once a certain amount of gas accrues in the test tube, it erupts out, forcibly disconnecting the tube from the stopper. And we have liftoff!
The stopper is secured in the tube such that force must be applied to remove it. Under normal circumstances, you’d easily apply this force using your hands. But how does gas do the same from inside?
Initially, the tube contains only air. And the air within and without the tube is more or less uniform: it consists of a large number of molecules, mostly nitrogen N2 and oxygen O2, which are constantly moving around. At room temperature, the average speed of such molecules is nearly 500 meters per second (1800 km or 1118 miles per hour!). That’s a very high speed; for comparison, F1 race cars travel at speeds 5 times less – up to 100 meters per second (360 km/h or 224 m/h)!
In other words, air molecules move quite quickly, colliding amongst themselves and with surrounding objects. Luckily, they are very small, so we don’t feel these collisions on an individual basis, but we’re familiar with their cumulative result – pressure. Pressure, which is usually associated with the P symbol, is a characteristic that describes how many of these molecules collide with an object per time unit, and how quickly these molecules are moving during these collisions. Even the masses of these molecules contribute to this calculation, but we will disregard this for now. We might even say that surrounding air molecules put pressure on us and on objects such as the tube.
While gas starts forming and accumulating inside the tube, conditions outside the tube remain relatively unchanged. After a period of time, there are many more gas molecules inside the tube hitting the walls and the cap than outside the tube. We say that there is relatively high pressure inside the tube. And at some point, this pressure grows enough to overcome the force (known as friction) holding the stopper secure in the tube. And so the tube launches!
You might obtain the same result sans chemical reactions by heating the tube (and, consequently, the air inside it). This will increase the gas molecules’ speed and make them collide with the walls and cap more vigorously, causing the pressure to rise.
The third way to make tube disconnect from the cap and launch is to lower the pressure outside the tube. We can accomplish this by placing the tube in a special vacuum box and removing the air from the box. Soon, there will be so few molecules outside the tube hitting the cap and tube from the outside, that the pressure inside the tube will be able to overcome the force of friction.
What is the gas we obtain and how does it form?
It is carbon dioxide CO2. Carbon dioxide forms when we mix sodium hydrogen carbonate NaHCO3 and citric acid.
When we turn the test tube upside down, the sodium hydrogen carbonate NaHCO3 and citric acid H_[3}C6H5O7 powders dissolve in the water and begin interacting. As they dissolve, the following ions form:
NaHCO3 → Na+ + HCO3–
H3C6H5O7 → 3H+ + C6H5O73–
H+ from citric acid and HCO3– from carbonate react to yield water and carbon dioxide gas:
H+ + HCO3– → H2O + CO2↑
Such a reaction is rather intuitively (as the substances quite literally exchange ions with one another) known as an ion exchange reaction.
Carbon dioxide gradually accumulates and noticeably increases the pressure inside the tube. As there is not enough space for the air and carbon dioxide molecules combined, they begin to push on the stopper and on the walls of the test tube. At some point, the stopper can no longer contain the pressure, so it disconnects and comes to rest at the bottom of the beaker. There is nothing stopping the test tube, though, which jets off like a rocket.
How does the tube fly? Do rockets fly the same way?
Actually, the tube flies rather like a cannonball shot from a certain angle into the air. Real rockets fly due to other physical processes that result when a huge volume of heated gas is released from the rocket nozzle. However, the driving force in a rocket also relies on another sort of chemical reaction known as an oxidation-reduction reaction.
How can I make my tube fly as high or as far as possible?
To make the tube fly as high as possible, place it vertically on its stopper, pointing the nose straight into the sky.
To make the tube fly as far as possible, place it at a 45o angle.
Why should you avoid mixing the reagents and water before the last step?
When dissolving in water, citric acid and sodium hydrogen carbonate react with each other immediately. This process yields quite a bit of carbon dioxide CO2, which should serve as the “fuel” for our rocket. However, a certain quantity of CO2 must accumulate in the closed test tube for the rocket to fly; if you simply pour all of the reagents into the water, much of the forming CO2 will escape before you seal the tube.
That’s interesting!
In order to fly, a modern jet rocket needs fuel. Normally, when we say “fuel,” we mean flammable substances. But what kind of fuel works best for rockets?
Rocket propellants, first of all, differ in their states of matter: they can be solids, liquids, or hybrids. Each of these states has its advantages and disadvantages.
The most widely-used propellant is liquid because liquid propellant allows for greater precision in maneuvering. Not only can an engine be turned on and off, but thrust can be adjusted accordingly. It works similarly to a gasoline-fueled car! Additionally, the liquid propellant is relatively inexpensive.
In an open space, however, liquid-fueled engines must be handled very carefully. Even their transportation requires caution. Needless to say, an accidental fuel leak onboard a rocket would be extremely dangerous! Moreover, in zero gravity, a huge volume of liquid can be unstable, which can, in turn, throw an entire rocket off balance. On top of it all, it would seem that we have already reached the limits of what can be done with liquid fuel. Unfortunately, it would be impossible to send a huge and heavy spaceship off on a distant cosmic voyage with kerosene propellant. A ship wouldn’t be capable of towing it along in such quantities. The same applies to the otherwise-convenient liquid oxygen and hydrogen: they can’t be compressed enough to be taken in sufficient quantities.
Solid propellants consist of two components: a flammable substance and an oxidizer that causes said flammable substance to ignite at the right moment. Both components are compressed so as to take up less space — and voila! Everything is confined, controlled, and safe! There is, however, one significant drawback: once a solid fuel is ignited, it will burn until depleted. In other words, there is no way to switch such a rocket “on” and “off”. Its maneuverability is limited as well.
The third type of rocket fuel is hybridized. At this point, it would seem the most promising version of the three. Its advantage lies in the fact that its components — a solid flammable compound and a gaseous or liquid oxidizer — can be mixed even in the ship itself. Thus, when necessary, the flow of the oxidizing component can be turned off to stop the engine. Moreover, hybrid propellants are quite safe and reliable.
The liquid component in a hybrid propellant is usually compressed oxygen O2. In everyday life, gaseous oxygen serves as an oxidizer when we grill meat or light a match. Nitrous oxide N2O is another perfect oxidizer, which is used to increase the speed of gasoline combustion in ordinary automobile engines.
The solid component (i.e. the flammable substance) can be anything that burns. Fuel used for space flights has to produce the maximum amount of energy per unit of mass burned. And it has to release this energy quickly — spaceships require powerful thrust. Yet, from a theoretical point of view, even paraffin or polyethylene can provide the needed thrust.
And from a practical point of view?
“MythBusters” took an experimental approach to this question. They used nitrous oxide as an oxidizer, and for their solid flammable compound, they chose… salami! Why? Salami is very fatty, and fat is combustible. Moreover, salami contains enough nitrates (nitric acid salts), which readily ignite and even explode. Many explosive mixtures contain nitrates.
Needless to say, these creative experimenters succeeded! Granted, their rocket’s path was short and crooked, but it took off nonetheless!